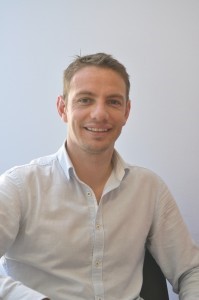
Cardiff University, UK
Materials for Neuroscience
We are interested in creating and analysing new materials (also nanoscale materials) for applications in the field of neuroscience and in particular neurodegenerative disorders. At present neurodegenerative disorders lack any disease modifying therapies. We are investigating whether materials such as scaffolds or soft gels could impact upon therapies for such diseases in the future.
We are also interested in developing materials to aid cell biologists and neuroscientists answer scientific questions deemed tricky to set up practically in the lab. For example we have developed hydrogels for live imaging of tissue culture and scaffolds for focal delivery to brain slice cultures.
Location
Currently, we are spread across two sites. I am based at Cardiff University in the School of Pharmacy and Pharmaceutical Sciences where I am building up a lab. However, due to my strong connection with the Leibniz Institute for Polymer Research, Dresden, I have a contract there and students working on cryogel scaffolds and microfluidics.
Current Projects:
Material 1 – Cryogel Microcarriers – together with Dr. Welzel (IPF, Dresden) we are developing highly porous hydrogel scaffolds termed “cryogels”. We are now investigating their use for a variety of applications. They are sponge like and can compress and re-expand to their original shape and size making them useful as an injectable scaffold.
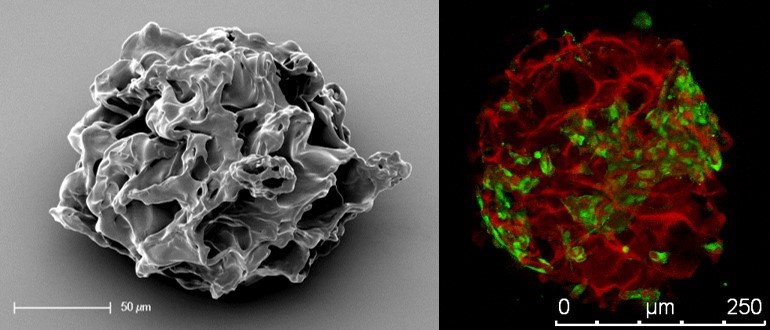
Microcarriers based on poly(ethylene glycol) and heparin. SEM image (left) and confocal microscope image of microcarrier (red) and PC12 cells (green) adapted from Newland at al., Small, 2015.
Dopaminergic neuron survival. Together with Prof. Anne Rosser (Brain Repair Group, Cardiff University) we are analysing if transplanting dopaminergic neurons on microcarriers improves their survival post transplantation into the Parkinsonian brain. The microcarriers are highly sulfated (so carry a negative charge), so we can use them to deliver heparin binding growth factors such as glial cell line-derived neurotrophic factor (GDNF) which may also help improve the survival of the graft.
Interleukin-13 (IL-13) delivery. The microcarriers also load and release IL-13, a protein that confers anti-inflammatory properties. We are investigating whether microcarriers can successfully deliver IL-13 to the brain to prime microglia to a more pro-regenerative/anti-inflammatory phenotype. If successful IL-13 loaded microcarriers may be useful for Multiple Sclerosis research.
Doxorubicin delivery. We also found that the microcarriers have a very strong affinity for loading the anti-cancer drug doxorubicin. They can uptake a large amount of the drug through electrostatic attraction and release it over a period of 40 days (in vitro). We would be interested to see if injection into a glioblastoma model (or resection model) would hold greater therapeutic potential than systemic drug administration.
Neural Progenitor Cell (NPC) Culture – an alternative to neurospheres? This is a small project performed in collaboration with Dr. Ehret (Prof. Gerd Kempermann lab) where we are investigating if neural progenitor/stem cells can be cultured on microcarriers for extended periods of time. We are interested to see if they hold their pluripotency and retain a healthy core even as they grow in size.
Material 2 – Cryogel Sponges – Cryogel sponges – in a similar fashion as described above, these are highly porous scaffolds. These are larger in size and shaped like a disc, for placing in cell culture inserts or ex-vivo cultures.
Focal Demyelination – Prof. Anna Williams (University of Edinburgh) has created an ex vivo model of Multiple Sclerosis by culturing brain slices and treating them with Lysophosphatidylcholine (LPC) which demyelinates the neurons. Her team has used our cryogel scaffolds to make this model better mimic the patchy nature of the human condition (pathalogical lesions surrounded by apparently healthy tissue). The cryogels could deliver LPC to a small region of brain and spinal cord tissue, close to where the cryogel was placed, meaning that most of the tissue is myelinated, but with a focal spot of demyelination allowing a better understanding of the demyelination/remyelination process. See a video description of the work and how this model reduces live animal use:
Material 3 – Hydrogel for live imaging – Dr. Katie Long is investing the effects of various extracellular matrix (ECM) proteins on the developing brain. The commercially available hydrogel that she was using for live imaging embedded tissue was composed of collagen so was not ideal for such purposes as it is comprised of an ECM component itself plus it had the problem of auto-fluorescence. We created an inert mixture without background fluorescence that allowed her to position the tissue at will, then set it into a hydrogel with similar mechanical properties to the human brain.
Material 4 – Polymer nanotubes – These are nanotubes largely composed of poly(ethylene glycol). They are soft and flexible and show no signs of toxicity (tested across 8 cell types in vitro). They may be a good alternative to carbon nanotubes for applications in focal drug delivery
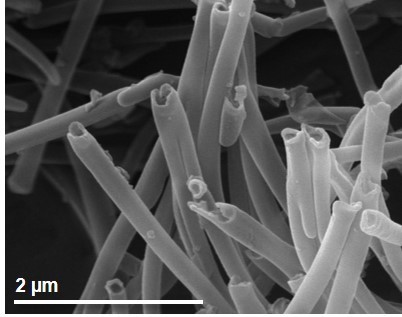
Poly(ethylene glycol) nanotubes (PEG nanotubes, not carbon nanotubes!) as shown by scanning electron microscopy. Adapted from Newland et al., Nanoscale, 2018.
Material 5 – Oxygen producing materials – We are creating a variety of oxygen producing materials that use either solid or liquid peroxides to generate molecular oxygen for applications in tissue engineering and regenerative medicine.
These are all in various depths of investigation, with varying degrees of success (or lack thereof in some cases!). More to follow.
Funding
We are extremely grateful to funders past and present including the Wellcome Trust (Sir Henry Wellcome Post-Doctoral Fellowship), Parkinson’s UK, Deutsche Forschungsgemeinschaft (DFG) and institutional support at Cardiff University via the School of Pharmacy, and the ISSF Wellcome Trust funds.